Science Meets History: Incident Analysis of H. L. Hunley
Background
H. L. Hunley was an experimental submarine built by Confederate civilian engineers during the Civil War. Powered by a hand crank operated by seven men with one pilot, the 40-foot long boat was built of wrought iron boiler plate with custom cast iron fittings. It was designed to submerge beneath an enemy vessel while towing a torpedo behind, which would strike the hull and detonate. After several fatal accidents, the military authorities working with the submarine in Charleston, South Carolina, ordered the boat be operated only at the surface, with a spar-mounted torpedo at the bow.
On the night of 17 February 1864, the crew, under the direction of Lt. George Dixon, left their base on Sullivan’s Island on the four mile journey to the outer ring of ships blockading the entrance to Charleston Harbor. Their target was the 1240-ton steamship USS Housatonic. Approaching silently in darkness, witnesses reported seeing something at the surface that resembled a floating log that was moving against the current. Raising the alarm, they began firing with rifles and muskets, but it was too late – the intruder made contact and, shortly thereafter, an explosion shook the Union vessel. Within minutes it was resting on the seabed. Five men were killed in the attack; however, most of the crew was able to cling to the rigging, which remained above water, until rescue arrived. From that vantage point, one witness reported seeing a light at water level in Hunley’s position. That is the last surviving potential sighting of the submarine and its crew of eight.
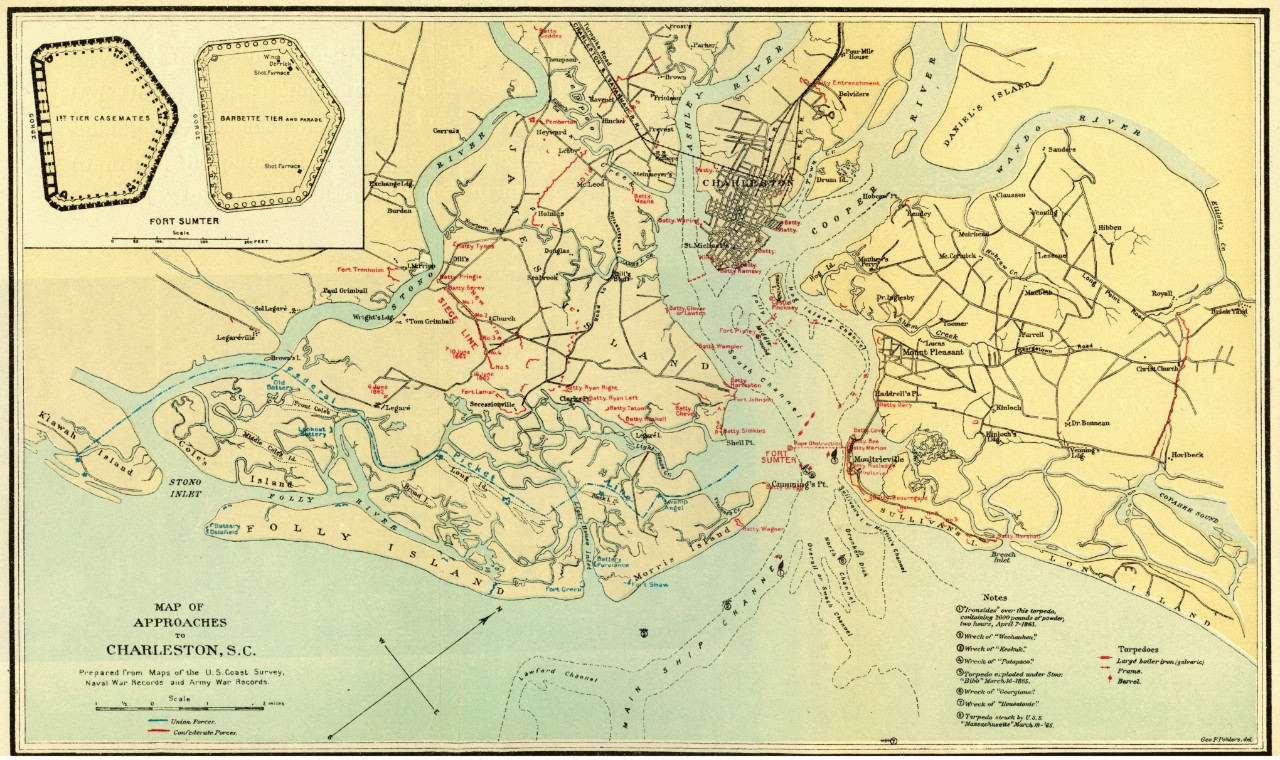
In the following years, many wondered what had become of the intrepid vessel, which had earned a place in history as the first submarine to sink an enemy vessel. In 1995 a survey team, working under the direction of author Clive Cussler’s organization NUMA, discovered the submarine buried under three feet of sediment less than 1,000 ft. from its victim. A Navy-led team of archaeologists and engineers raised the submarine and its contents in 2000, installing it in the custom-built Warren Lasch Conservation Center (WLCC) in North Charleston for excavation and conservation.
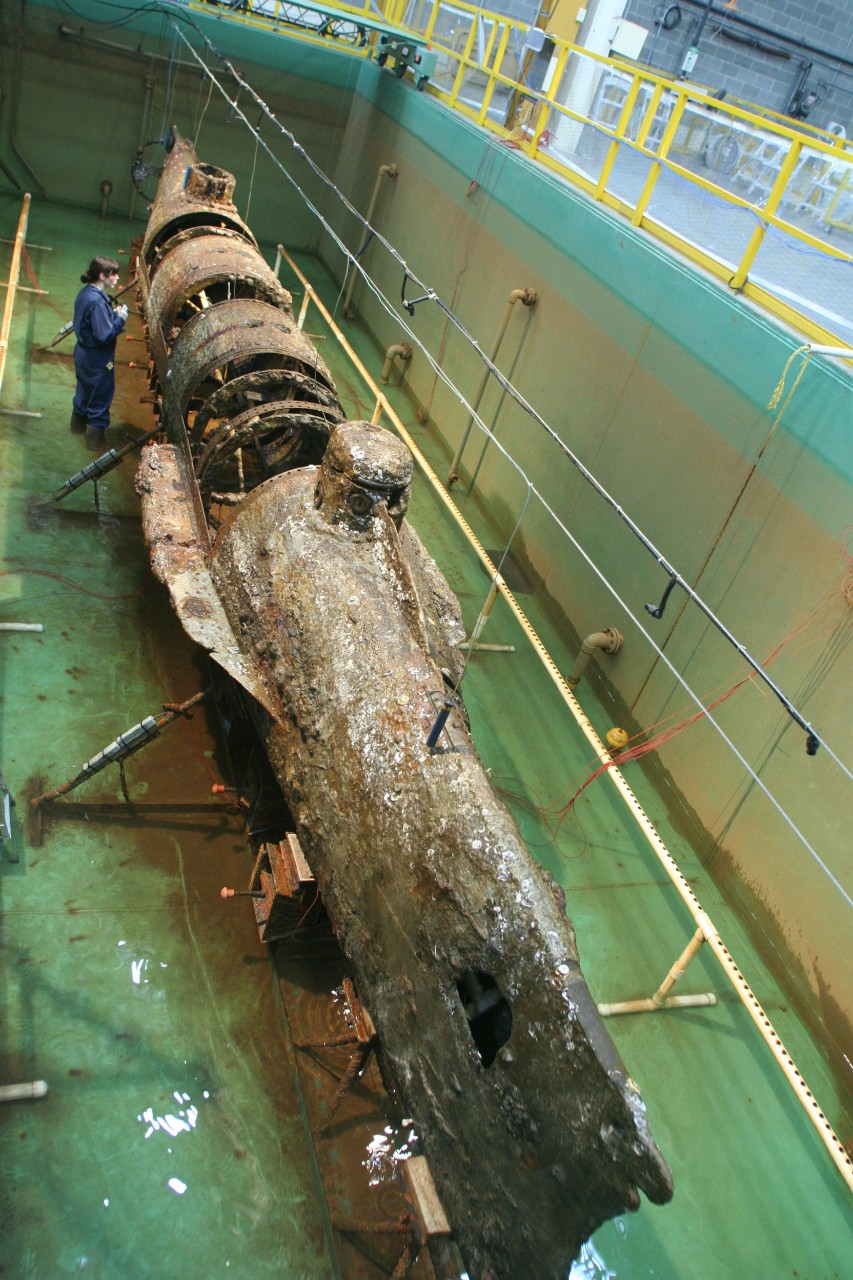
Questions
One of the first things researchers noted upon recovery of the vessel was that there did not appear to be any damage to the hull serious enough to have caused its loss. There were two significant holes on the starboard side that had clearly developed well after the sinking, as water-borne sand scoured away at the exposed iron. One additional hole was found in the forward conning tower that did appear to have occurred during or soon after the attack on Housatonic. However, if the vessel was running at the surface, as reported by witnesses, this area would have been above the waterline and should not have led to catastrophic flooding.
Archaeologists learned a great many interesting things about the design of the submarine and the lives of the people on board, but they still could not explain why the boat sank in the first place. Many people, both professional and avocational, put forward suggestions. Could the explosion have led to hull distortion allowing water in at the seams? Was the crew incapacitated by the explosion effects? Was the vessel so unstable that taking on a small amount of water from the conning tower hole could become an unrecoverable event?
At first, it seemed these questions could not be answered, as there were too many unknown variables. However, two discoveries changed that. The first was a document discovered in the National Archives by Mark Ragan, a researcher for the Friends of the Hunley. This was a diagram of a spar-mounted explosive device labeled “Singer Torpedo used for blowing up the Housatonic.” It provided the remarkable statistic that this torpedo held 135 pounds of black powder – a significant increase over the 90 pounds reported in an account from 1902.
In 2012, a second discovery filled in another essential variable. While deconcreting the tip of the iron spar, WLCC conservator Paul Mardikian found that a portion of the torpedo’s copper sheathing was still bolted in place. This contradicted contemporary accounts, which had suggested that the submarine had somehow affixed the torpedo to Housatonic’s hull and then backed off before detonating it. Now researchers knew the exact distance the submarine was from the explosion – a mere 16 feet.
In order to determine the impact of these findings, Maria Jacobsen, then lead archaeologist at the WLCC, and Dr. Robert Neyland, head of NHHC’s Underwater Archaeology Branch, reached out to Naval Surface Warfare Center Carderock Division (NSWCCD), the Navy's primary lab for research, engineering, modeling and testing of ships and ship systems, for technical assistance. Perhaps NSWCCD engineers could help interpret the events of that historic night in 1864?
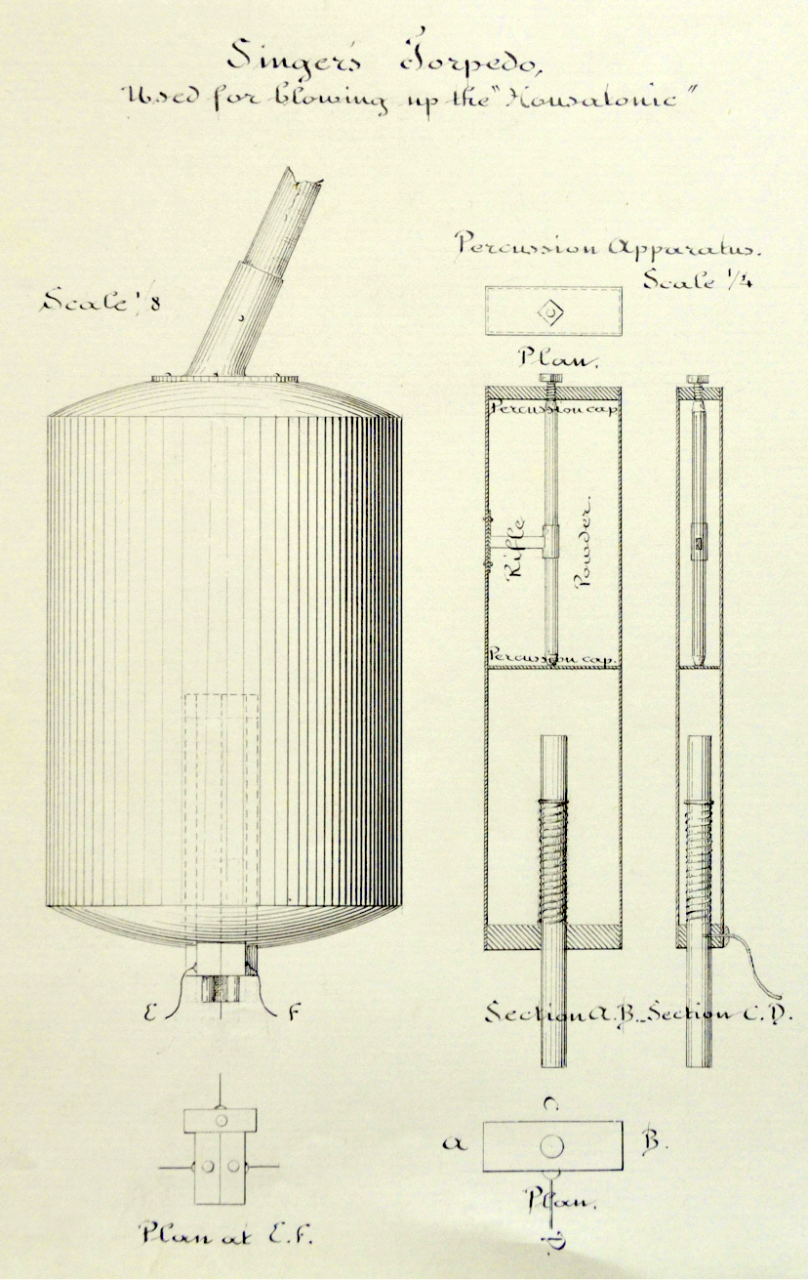
Carderock Takes the Case
After an initial meeting at NHHC’s office in the Washington Navy Yard in 2013, Carderock’s answer was a resounding yes. A project was initiated the following year by Dr. Ken Nahshon, of Carderock’s Hull Response and Protection Branch, to leverage and apply the extensive capabilities of the Navy’s most advanced in-house modeling and simulation (M&S) tools to help answer what might have happened to Hunley and its crew. In the process, those working with the M&S tools would be afforded the opportunity to develop models from data collected from a variety of sources, including historical records, laser scans, and environmental data, as well as to evaluate the underwater explosion (UNDEX) behavior of propellant materials for incorporation into future models.
Dr. Nahshon’s team determined early on that key technical challenges to performing his work would include developing accurate virtual structural representations from archeological measurements, capturing the effects of the explosion and crew response, and most critically, determining the UNDEX output of black powder itself.
A parallel study was initiated to evaluate what might have happened to Hunley in the aftermath of the explosion, by examining the power, weight, stability, and drift characteristics of the submarine. This work was led by Prof. Matthew Collette of the University of Michigan’s Marine Structures Design Laboratory who has worked extensively on other Navy projects with Dr. Nahshon and others. The results of both studies, when put together, would prove critical in establishing a complete picture of the sequence of events from the moment of the explosion until Hunley reached the sea bottom.
Realizing the unique combination of challenging technical problems and impact that the analysis findings could have on naval history, Dr. Paul Hess, Program Officer at the Office of Naval Research’s (ONR) Ship Systems and Engineering Division, and Dr. Jack Price, Director of Research at NSWCCD made early and continued investments in the program. Later explosive characterization efforts performed by NSWC Indian Head EOD Technology Division (NSWCIHEODTD), which focuses on the research, development, testing, evaluation, and in-service support of energetics and energetic systems, were funded by Dr. Michelle Skoorka, Program Manager for ILIR at ONR, and Dr. Alfred Stern, Director of Research at NSWCIHEODTD.
Explosion Modeling
Carderock’s M&S calculations were performed using both the Navy Enhanced Sierra Mechanics (NESM) and DYSMAS software programs, whose development was led by Carderock and Indian Head, respectively. Both software programs directly capture the fully coupled response of UNDEX and structure interactions. This is achieved through two separate software codes that talk to each other: a software code that captures structural motions, deformations, and loads, talks directly to a second software code that captures the response of fluid regions including the water, black powder, and air. While DYSMAS/FD, the fluid code common to both NESM and DYSMAS, was fully capable of capturing the loading correctly with some minor modifications, the lack of black powder UNDEX data meant that physical testing of black powder would be required to have confidence in the M&S results.
In order to capture the response of the crew-members themselves to the explosion, numerical models of automotive “crash dummies”, or anthropomorphic test devices (ATDs), were subjected to the numerically simulated UNDEX loads using the commercial software code LS-DYNA. This approach was first performed under ONR’s Human Injury & Treatment (HIT) program and performing the current investigation and addressing many technical issues would turn out to advance the Navy’s capability to capture crew response to UNDEX. Based on the known heights of the crew derived from studying their skeletal remains, the 50th Percentile Male ATD was selected – these are 5’ 9” tall and 171 pounds. Interestingly, it was discovered that the men could not have fit behind the crank shaft in a normal seated position with full clearance for the handle to pass their knees, and likely had to stand in an arched position while turning the crank.
A key aspect of successfully performing the calculations was the availability of substantial High-Performance Computing (HPC) resources that were generously provided by the DoD High Performance Computing Modernization Program (HPCMP).
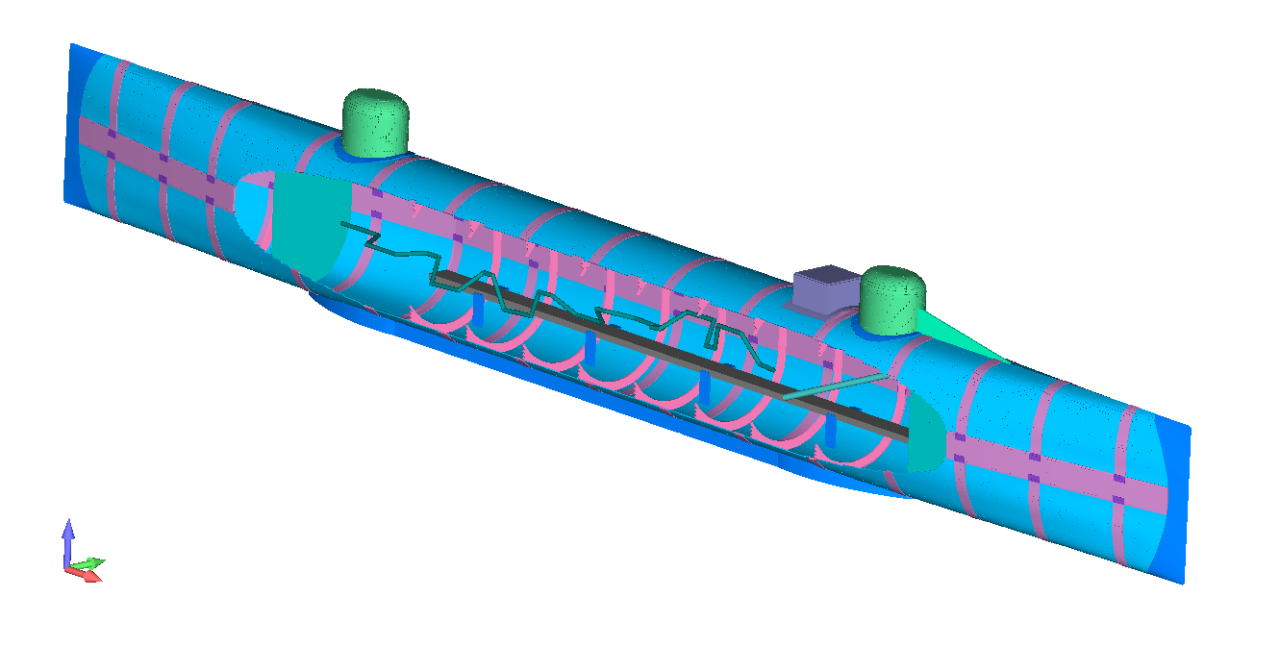
Numerical Model Development
Now that the technical approach was determined, work began to prepare for the calculations. Although the available M&S tools are extremely powerful, they require a tremendous number of inputs ranging from detailed weights of the vessel and plate thicknesses to the curvature of the hull. Fortunately, most of these details were readily available due to the work that WLCC had performed in documenting Hunley. Laser and structured light scans conducted at the WLCC under the direction of archaeologist Michael Scafuri were generously made available. The scans, which consist of millions of physical distance measurements that fully capture the shape of Hunley’s hull in a digital format, would prove invaluable to both Dr. Nahshon and Prof. Collette’s efforts in developing an accurate virtual representation of the submarine. In order to capture USS Housatonic, a 3D numerical model developed by WLCC from archived drawings of its sister ship, USS Ossippee, was utilized.
Black Powder Testing & Model Development
With an accurate virtual structural representation in hand, the next step was developing inputs for DYSMAS/FD able to describe the explosive output of a black powder UNDEX. While extensive characterization of black powder exists in smaller quantities applicable to firearms, the only UNDEX data of relevance dated back to tests in 1919. This data would prove useful for initial calculations, but unfortunately it only described the initial emanated pressure wave and contained no information on the subsequent explosively-generated bubble, a potentially damaging characteristic of underwater explosions. Since much of the complexity of capturing black powder’s output results from its nature as a deflagrating propellant material rather than a military-grade high explosive such as TNT, this older data served as a tantalizing clue as to what testing would reveal.

Since black powder’s explosive reaction is highly sensitive to confinement and grain size, testing efforts were focused on performing full-scale tests on representative explosive charges that would fully capture the behavior of Hunley’s torpedo. Testing was accomplished by a highly experienced team of experts, including staff from Carderock’s Dynamics Measurements Group, led by William Lewis, as well as Dr. Thomas McGrath and Gregory Harris from Indian Head. Working together with test personnel and technicians at Aberdeen Test Center (ATC), a series of six UNDEX tests was conducted at the Underwater Test Facility (UTF) in late 2015. Tests were conducted at an 8-foot depth mimicking the engagement and at a 50-foot depth to capture the oscillating behavior of the explosively-generated bubble. Multiple sensors were deployed at different depths and distances from the explosion to record its effects, resulting in the high resolution data that was required.
Working with this newly obtained data, Dr. McGrath at Indian Head generated a new numerical model of the explosive reaction of black powder suitable for use in DYSMAS/FD. This model proved to be of extremely high fidelity in capturing both the recorded pressures and bubble phenomena measured during testing, thus providing a high level of confidence in the numerical simulations of Hunley’s response to the explosion.
High speed video footage of the testing of an explosive charge mimicking H. L. Hunley's torpedo.
The charge casing, black powder fill, and depth are consistent with the most recent understanding
of the
submarine's attack on Housatonic.
Summary of Findings
With all the required inputs in hand, Carderock performed the numerical simulations of the explosion, the imparted load to Hunley, and the loads imparted by Hunley’s hull to the crew. Most critically, it was found that while the loads to Housatonic would have been devastating, the loads to Hunley are relatively modest, despite the small amount of standoff to the explosion. This is a result of the nature of black powder, namely that the initial pressure pulse emanating from the explosion, while long in duration, is of relatively low pressure. However, black powder is highly effective at generating a bubble of gaseous explosive products. This bubble oscillates in size and finally collapses on itself resulting in a large high-velocity slug of water, or bubble jet, able to punch right through steel, let alone Housatonic’s wooden hull. Due to Hunley’s location, the submarine would have been just far enough away to not be impacted by this jet. It was observed that the primary response of Hunley to the explosion was a rapid vertical motion resulting from the flow of water around the bubble.
Simulation of the underwater explosion from H. L. Hunley's torpedo attack on USS Housatonic,
illustrating the bubble expansion and collapse.
The response of the crew to this stimulus is effectively captured by the ATD. It was found that the change in velocity experienced by the ATD in the simulation was not enough to induce skeletal damage, which is consistent with the findings of the Smithsonian Institute’s Dr. Doug Owsley, who studied the human remains excavated from the submarine. While the occupants may have experienced some bumps and bruises, it does not appear there was enough force to cause concussion or other forms of complete incapacitation.
Simulation of the crew response to the impact of the explosion on H. L. Hunley
Flooding, Stability, and the Ability to Escape
Working in parallel, Prof. Collette and his students examined the vessel’s ability to float with differing levels of damage, and to escape from the scene. In order to develop an accurate understanding of Hunley’s stability, power, and weight, computer models had to be developed describing the hull and internal components. Creating these models for a historical submarine proved to be a complex undertaking as a result of the limitations of working with an active archaeological project as well as the degraded state of Hunley’s hull. One of the difficulties was that many of the interior components of the vessel were still attached and could not be weighed independently. Thus, their weights had to be calculated based on volume and material density. The students carefully cataloged each item on the vessel, locating its center and estimating its mass. Using a digital hydrostatics model of the vessel, virtual stability tests were then performed, showing that the boat could right itself well when operated at the surface, but that, when submerged, is was very sensitive to forward and aft weight shifts. Additionally, simulated propulsion tests and a scale physical model tested at the University of Michigan’s towing basin revealed that the vessel could cruise for long distances at slow speeds near 1-2 knots and could sprint for short distances at 3-4 knots.
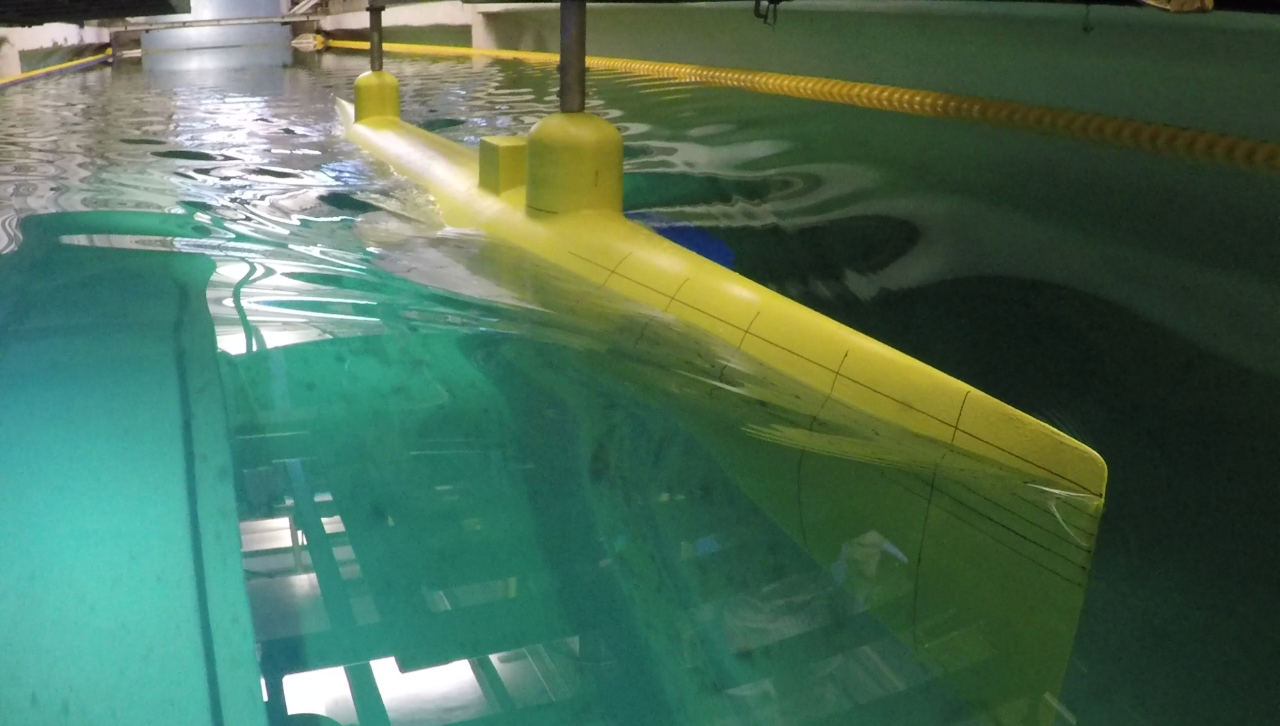
The hydrostatic model also showed how the addition of water, either through filling the ballast tanks or from leaks in the hull, would have affected Hunley’s performance. Prof. Collette and his team found that the addition of between 50 and 75 gallons of water would sink the vessel when Hunley was ballasted for running on the surface. This fact was critical in examining a piece of evidence revealed only when conservators at WLCC began deconcreting the outer hull – a broken outlet pipe in the forward ballast tank. The pipe was cracked at the juncture with the hull plating and offset allowing uncontrolled water flow into the crew compartment. Using photographs, measurements, and laser scan data, a replica of the damaged pipe and the section of the hull near the breach was created using additive manufacturing, or 3D printing. The configuration was then tested at several submergence depths to determine how quickly the water would enter the vessel. The tests revealed that the vessel would sink in approximately 3 minutes from the initial failure of the pipe.
Knowing how long the vessel likely remained afloat, the team examined drift models based on the probable speed and direction of current taken from historical records. If Hunley were drifting under no power, it would have taken approximately 13 minutes to reach a point 1,000 ft. from Housatonic. Given that this is much longer than the time necessary to sink the vessel, it suggests that something more than just the pipe alone may have been responsible for the loss of the vessel. Perhaps some of the crew were still turning the crank to move the vessel, or attempting to stem the flooding. At the vessel’s higher sprint speed, it could cover the estimated distance in time. However, if the crew was able to maneuver the vessel, why did they make no effort to escape? Thus, while we have learned a great deal more about the operation and capabilities of the vessel, the mystery around the events of February 17th still remains.
Conclusions
All of these findings have significantly refined our understanding of the events that took place the night of Hunley’s loss. The project provided valuable opportunities to advance the Navy’s capabilities to characterize the explosive output of non-conventional explosives, perform advanced simulations in support of forensic analysis of a weapon attack on a ship with limited data, and integrate the impact of explosions into computational simulations of crew. These lessons learned and technical output from this project have already been transitioned into use in other Navy programs.
Many of the University of Michigan students enthusiastically embraced the Hunley project and have found the historical mystery particularly compelling, leading to the development of additional research questions for future studies. This fusion of the technical and the cultural has provided a promising model for further collaboration both within Navy and with external research partners.
While this project has generated substantial new knowledge with regards to Hunley’s sinking, there is still more to the mystery that requires further exploration!
Acknowledgments
This project would not have been possible without the generous support of Dr. Paul Hess, Dr. Jack Price, Dr. Michelle Skoorka, and Dr. Alfred Stern. The dedicated support of Dr. Ken Nahshon and the project team at NSWCCD, NSWCIHEODTD, and ATC has been phenomenal. Dr. Collette and his team at the University of Michigan have done amazing work and brought a high level of enthusiasm and motivation to the project.
The cooperation and assistance of the dedicated scientists at the Warren Lasch Conservation Center at the Clemson University Restoration Institute, including Stephanie Cretté, Michael Scafuri, and Paul Mardikian, was indispensable and much appreciated.
Finally, Maria Jacobsen’s initiation of this project is gratefully acknowledged. Without her, this project and all the technical work that has been accomplished would have never occurred.
Further Reading
About NSWC Carderock Division
About NSWC Indian Head EOD Technology Division
About the Aberdeen Test Center
About the Marine Structures Design Laboratory at the University of Michigan
About the Clemson University Restoration Institute